I love the invention and design development process. I love seeing the concepts come together, love working through iterations of prototypes and finally (finally!) getting a design just right, fulfilling all the technical and aesthetic requirements. But do you know what’s even better? Seeing your designs in full-scale manufacture.
Unfortunately, once your design is beautiful and working well, that’s only half the battle won. For most plastic parts, you’ll need the design to be injection molded, which is the most common low-cost technology for producing large numbers of plastic parts. (Rarely, for very specific geometry, parts are milled with CNC, but that usually costs 50-100 times as much per part.) And that comes with its own set of specific requirements, or you could be looking at $100,000 molds for your parts. But never fear! With the design guidelines below, you can keep tooling costs low and still have your design looking as beautiful as ever.
But before we get into the specifics of how to adapt your 3D printed design for injection molding, let’s first take a look at the manufacturing process: how does injection molding work?
Injection Molding Basics
At its core, injection molding is simple: two large metal mold halves come together, a plastic or rubber material is injected into the cavity they form, it all cools, and then the part pops out while the two halves separate again. Repeat ten thousand times, and you have your first run of parts ready for marketing. (Quick note on terms: The direction in which the molds move is called the “mold pull”, while the line around the part where the two halves meet is called the “parting line”.)
In practice, it can get more complicated. Generally, even though the plastic materials being injected are melted, they aren’t really heated: The material is pressed into the injection ports (called gates) via a large auger screw. As the material is compressed, it heats and begins to flow into the molds.
And the molds themselves have to be “negatives” of the part being molded, and that geometry can get quite complex. If you look at a very simple red cup, the kind we all played beer pong with in college, you can see how the interior of the cup would be formed with geometry from one half of the mold, while the exterior is formed from the other half. That’s about as simple as it gets, and already you have a large cone of material from one side protruding into the other.
Simple red cups are designed for ideal injection molding (Image Credit: Arvind Grover)
As the shapes being produced become more complex, the molds get more complicated, too. Instead of the classic red cup, imagine a simple plastic coffee mug. The interior comes from one mold, the exterior comes from another—but wait! What about the handle?
When part geometry can’t be formed from the two molds coming together in a straight line, then the molds will need to have “action”: “slides” (pieces of the mold that come in from the side, like in this video) or “screws” (parts of the mold which twist into place). With mold action, very complex shapes can be formed, including threads for nuts and screws. But this versatility comes at a price: Often there is a 10X difference in price between simple “straight pull” molds and those with action.
And with that kind of a price tag on fancy mold tooling, it pays to take the time to design your parts for easy molding. What geometry will cause problems, and what designs will make your chief accountant break out into that endearingly nerdy smile of his?
Preparing Your Design for Easy Molding
The biggest rule for reducing cost in mold tooling is this: Avoid undercuts. Undercuts are when one part of the geometry is underneath another, when that part of the geometry can’t be formed with either mold half or will keep the part stuck in the mold.
For example, look at a common feature: snap fits. The snap fit on the left can’t be formed by a simple injection mold in this case because the underside of the snap fit isn’t accessible from the bottom—the other part of the case is in the way. To fix this, we can cut away a little material from the case, as in the picture on the right, and then the snap can be formed easily.
When I evaluate my designs, I often imagine the piece is on a glass table, with one light shining from underneath and another light shining from above. If the lights touch every surface of the piece, then it’s easy to mold; if any area is in shadow, then it’s an undercut, and I’ll find a way to redesign that area.
Another thing to think about in molding is how easily the part will release from the mold once it’s cooled and the molds separate. Several geometries will keep the parts from releasing easily, making molding difficult, and increasing the cycle time, and ultimately the per-part cost.
Whenever possible, if you have straight sides on your part that are in the same direction as the mold pull, you’ll need to add a small amount of draft or taper to the part. Like with the red cups, having the parts taper a little makes them release from the mold more easily, and when appearance is critical, that taper can be as little as one to two degrees, which is barely noticeable, but the longer the straight sides are, the higher the draft angle will need to be.
Similarly, sharp corners tend to get stuck in the mold, and so you should add fillets to all the corners of the molded part. The one exception is when the corner is also the parting line (where the two halves of the mold come together); because the corner is formed by two different parts of the mold, you can keep that corner sharp.
Between the injection and the ejection is cooling, and that dictates more part geometry. The part will start to cool very quickly once the cavity is filled, and the cooling starts from the outside, forming a skin on the material almost immediately. As the part cools, it shrinks. If all areas on the part are of similar thickness, then the part will shrink uniformly and looks good when it pops out.
However, if the part has some very thick areas and very thin areas, then the thin areas will cool and harden first, while the thick areas are still cooling. Because the thin areas surrounding this geometry are already cool, and a skin has already formed on the surface of the part, as the molten center cools and shrinks it will suck the surface down, causing sink marks—unappealing divots on the smooth surface of your part.
To avoid sink marks, keep the thickness of material relatively uniform throughout your part, no more than a ratio of two to three between the thin and thick sections, and don’t transition suddenly from a thin to thick area.
Finally, when you’re designing your parts, try to visualize which surfaces will be formed by the top mold half, which surfaces will be formed by the bottom mold half, and exactly where the parting lines will be when these mold halves meet. This takes practice to do well but makes the molding process substantially easier.
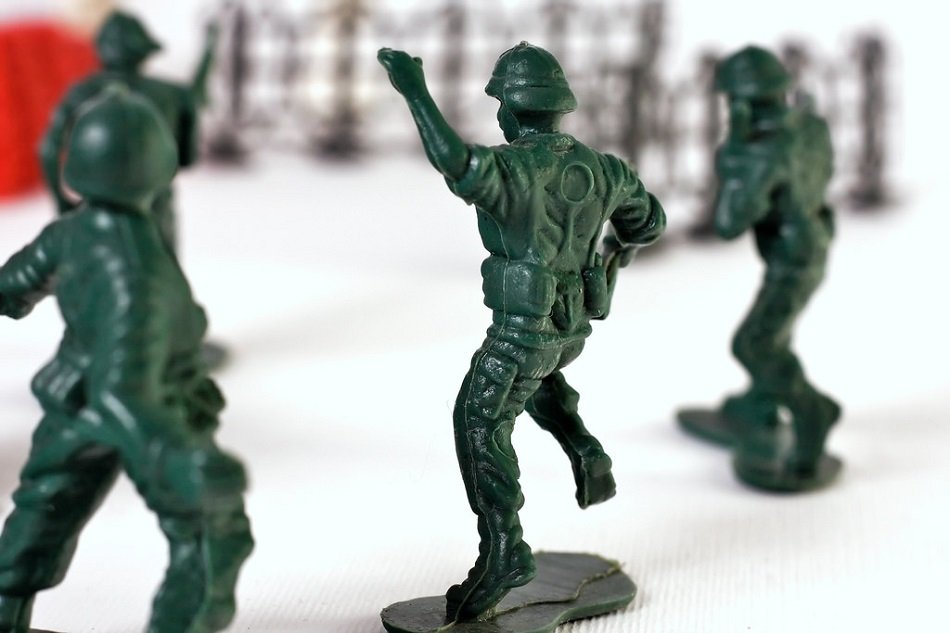
Green plastic toy soldier wearing World War II military gear and simulating the fall after being shot. Next to it there’s another one throwing a grenade into the enemy camp. In the background there are two barb wire fences.
The line on the side of this army man clearly shows where the two halves of the mold came together—that’s the parting line for the part.
While the design rules above will cover most of your part designs, sometimes products are too complex for simple molding in a single piece, and for those designs you’ll need to look at…
Sectioning Complex Geometry
Take a look at the picture below, a golf swing training device (the “swing coach club”) I helped a client design a few years ago. Obviously, with the hollow interior going in a different direction than the shaft mount, this wasn’t going to be an easy part to manufacture. Worse, inside the club head is a latch for the ball cradle (the blue piece), and the lines across the face are undercut. So how did we prepare the design for injection molding? Sectioning.
Instead of trying to mold the entire head as a single piece, we cut the head geometry into three separate pieces, which are then ultrasonically welded together after molding. The bottom and shaft mount of the head are one piece, along with the latch for the cradle, and the mold pull direction is the same as the shaft. The face, along with the cut lines and the mounting cylinders for those weights along the outside edge, are a separate piece, with the mold pull perpendicular to the face, while the top of the shell is a third piece.
While sectioning pieces this way can seem like a complicated design problem, this is often the only way to get a design to have both the right aesthetics and function. And even though we have three pieces instead of one, the tooling and molding is still less expensive than the cost of designing a mold with the dozen different types of action the design would otherwise require.
Obviously, though, when you make changes this drastic to your design, you’ll have to make new prototypes to test out the new geometry, which brings us to…
Testing Your Updated Designs with 3D Prints
Again, you ask? I know, I know! You thought you were past this part. But inevitably, as you alter your design for easier molding, you’ll end up making significant changes which will require further prototype testing. In addition to the changes above, you’ll also need to make sure that you’ve allowed clearance between assembled or sectioned parts, so that they come together smoothly.
For testing all this, I recommend using material as close to molded as possible, especially considering tolerances and surface finish. Most injection molded parts come within 0.010-0.020 inches of nominal (though you can specify closer, more expensive tolerances) and have smooth surface finishes. The closest approximation for these conditions is PolyJet printing, and the variety of plastics available means you can choose a material that will closely approximate the strength and stiffness of your final parts.
With your geometries adjusted and tested, you can move directly to injection molding. But most injection molding companies will require initial runs of 5,000 to 10,000 parts, and you’ll have to pay anywhere from $2,000-$20,000 per mold (for each piece) before you can get started. What if you’re not yet ready to commit to an order that large?
Low-Volume Production
Between the stages of prototype testing with 3D prints and full-scale manufacturing lies a happy medium: urethane casting. The process is similar to injection molding—two mold halves come together, the material flows into the cavity, and then the part pops out—but with room temperature materials and soft molds. However, the big difference is in the cost of tooling and the size of manufacturing runs: Tooling will often be one tenth the price, and you can do runs of parts as small as you like (typically in the 20-500 piece range).
When you’re preparing parts for urethane casting, all the design geometries are the same, and so many companies have used urethane casting for decades as a way to test out part in pilot builds before committing to the full tooling and production costs of injection molding. These days, with so many products going through crowd funding, urethane casting can allow you to satisfy your backers with real parts, without needing to set an enormous funding goal. Check out the Fictiv urethane casting info page for more information.
Stepping into the World of Injection Molding
So now you’ve adjusted your design for easy injection molding, sectioned apart the pieces which couldn’t be adapted, tested out all the new designs in a high-quality print, and even run a pilot of the new design in urethane casting—what’s next? Now, you’re ready to run with the big dogs: It’s time to get quotes on injection molding your design. And as luck would have it, that’s the topic of my next article—sign up here to be notified as soon as it comes out!